|
 |
Energy is vital to dynamic
societies and healthy economies. Consider the effects of the August
14, 2003, blackout, the largest in the history of North America. Within
15 minutes of the initial outage, five power grids and more than 50
million people — from southeastern Michigan through Ontario and
from northern Ohio to New York City — were in the dark. Many
were without power for three days.
This
was not an isolated incident; the power problem is a global one. In
June 2003 Italian electricity firms ordered the first nationwide power
cuts in more than 20 years. A year later China faced one of its most
severe power shortages since the 1980s. In fact, several provinces
imposed brownouts in an attempt to conserve energy. For the last several
years Scottish and British officials have been warning that, without
a significant investment to build power supplies and infrastructure,
blackouts similar to the August 14 event could occur in their countries.
In
the United States as politicians and power companies struggle with
technical concerns, consumer confidence, and ethical issues — such
as the roles of managers and regulators in the power industry — researchers
in the Notre Dame Energy Center are studying cost-effective ways to produce
clean, safe, and renewable energy, in order to lessen the country’s
dependence on foreign oil and other fossil fuels and reduce greenhouse
gas emissions.
The energy center was established in November 2005 to develop
new energy technologies. According to Center Director Joan
F. Brennecke, the Keating-Crawford Professor of Chemical and Biomolecular Engineering,
the center also hopes to play a key role in energy education and literacy,
the development of energy policy, and the exploration of the ethical
implications associated with energy. Notre Dame researchers have a proven
track record in energy related research, but with the creation of the
center, they join many universities across the country actively pursuing
clean energy technologies. “It
is such a huge challenge,” says Brennecke, “that we cannot
afford to have just one place working on it.
We need the full force of
our scientific and engineering expertise focused on this issue.”At
the same time, Brennecke stresses that the energy center cannot physically
tackle all of the challenges associated with energy. Instead, the center
will target five areas in which University researchers have expertise:
energy efficiency; clean coal utilization; carbon dioxide (CO2) separation,
storage, and usage; safe nuclear waste storage; and renewable energy
sources.

The concept of being more efficient with energy sounds simple.
After all, choosing an energy efficient light bulb for a floor lamp isn’t
exactly rocket science. But for those interested in conserving energy,
it’s as important as turning down the temperature of a water heater,
upgrading leaky windows, insulating hot water pipes, or driving a hybrid
vehicle. These and other “mundane” actions may seem insignificant
when considering the global energy picture, but a commitment to using
energy wisely while developing advances in energy efficiency, and other
viable energy technologies, is vital in both the short- and long-term.
It is especially important if governments, industries, or consumers think
they can rely indefinitely on fossil fuel reserves. According to Brennecke,
if the usage of fossil fuels were to remain constant (zero population
growth with no increases in usage), oil supplies would last for approximately
35 years, natural gas for 60, and coal for 400.
Engineers at Notre Dame
and around the world have been looking for ways to extend fuel reserves
by developing more efficient ways of generating power. For example, fuel
cells, which function like batteries, are inherently more efficient than
combustion power cycles and can generate electricity up to two times
more efficiently than a traditional power plant. Unfortunately, because
most fuel cells use platinum and other expensive catalysts, they are
not cost competitive. Durability is another issue. Fuel cell systems
need to be as robust and reliable as combustion engines to be effective.
In
projects supported by the U.S. Army and the Indiana 21st Century Research
and Technology Fund, College of Engineering researchers are targeting
new and less expensive materials for methanol (direct methanol) and hydrogen
(polymer electrolyte membrane/ PEM) fuel cells. By changing the flow
patterns within a PEM fuel cell (the units most often used in vehicles),
they have already achieved a dramatic increase in the efficiency of a
cell.
University researchers working in conjunction with the Department
of Energy are also attempting to capture the unused heat generated from
power generation systems (industrial cycles) and use it to provide energy
efficient absorption refrigeration. |
 |
According to an Associated
Press report dated April 29, 2006, “Taken together Exxon,
Chevron, and ConocoPhillips made a profit of $8.19 for every $100
in sales. In contrast, Internet bellwethers Google Inc, Yahoo Inc.,
and eBay Inc. collectively turned a $19.20 profit on every $100
of their combined revenue.” So why aren’t politicians,
consumers, and industry leaders clamoring to impose higher taxes
on these Web giants too?
Perhaps the difference lies in the fact
that society does not need the Internet to survive, in spite of
what teenagers believe. It does need energy. But the blame for
the energy dilemma cannot be laid solely at the feet of the oil
giants. The Energy Information Administration (EIA) estimates that
gasoline accounts for approximately 17 percent of the energy consumed
in the United States. Likewise, even though the demand for electric
power in the U.S. has risen by 30 percent in the last decade, while
transmission capabilities have grown by only 15 percent, power
shortages cannot be attributed only to power companies.
Could it
be that Americans are energy hogs? The EIA’s Annual
Energy Outlook estimates that the average amount of energy used per
person will continue to increase through 2030. Commercial energy
use is also expected to rise, being affected largely by economic
factors and population trends. Think about it: As Generation Xers
age, they accumulate more disposable income, which is spent in hotels,
restaurants, stores, theatres, and for transportation. In the ever-growing
information age, there is also more need for electrically powered
devices.
The truth is that a discussion of energy policy is long overdue,
yet changes would be unlikely to have an immediate effect since consumer
and industry behavior is part of the problem. Consumers seem reluctant
to scale back their usage, and industry is unlikely to make changes
that negatively affect the bottom line without federal intervention. |
 |
After
almost five years and hundreds of hours of debate, the 1,700-page
Energy Policy Act of 2005 was passed by Congress in July 2005 and
signed into law a month later. Some of the provisions in the act,
developed to combat growing energy problems, include:
— A
tax credit of up to $3,400 for the owners of hybrid vehicles.
— A
three-fold increase in the amount of biofuel (typically ethanol) to be mixed
with gasoline sold in the United States.
— The authorization of
$200 million annually for the development of clean coal technologies.
— The establishment of federal reliability standards
regulating the nation’s
electrical grid.
— Department of Energy authorization to build up to six
new nuclear power plants. |
|

A complex chemical substance,
coal contains carbon, hydrogen, and oxygen, but it can also contain
small amounts of nitrogen and sulfur, as well as heavy metals like
mercury. There are four types of coal: anthracite, also called hard
coal, which has the highest heating value; bituminous, or soft coal,
used mostly for electric power generation; subbituminous, used for
generating electricity and process heat; and lignite, which has a high
moisture content and low heating value. Lignite is also used to generate
electricity.
Coal has played a huge role in the development of the U.S.
In the 1300s the Hopi Indians used coal for cooking, heating, and firing
pottery. The first commercial mining of coal occurred in Virginia in
the 1740s. In the early 1800s it was used to make glass and replaced
wood as the fuel of choice for the first commercially practical American-built
locomotive, the Tom Thumb.
Produced at one time or another
in nearly all of the states that have deposits, coal has been used for domestic
heating, railroad fuel, iron smelting, and electricity. Today, more than 20 percent
of all of the energy consumed in the U.S. (and more than half of all of the electricity)
is produced by coal. It is by far one of the country’s most abundant sources
of fossil fuel, and the stage is set for it to once again power the economy.
In order to better address concerns about gases produced from burning coal, researchers
in the energy center are studying hydrogen-oxygen combustion and the catalytic
conversion of environmentally undesirable by-products of combustion, such as
nitrogen oxides (NOx) and sulfur dioxide (SO2). Sometimes seen as a reddish-brown
layer of air above urban areas, NOx contributes to the formation of acid rain
and nutrient overload that can deteriorate water quality. A significant contribution
to acid rain is also made by emissions of SO2.

Researchers are also exploring
ways to capture and store the CO2 produced when coal and other fossil fuels are
burned, instead of releasing it into the atmosphere. One of the most promising
processes involves the use of ionic liquids for flue gas and coal gasification
separations.
Ionic liquids, organic salts that are liquid at room temperature,
easily absorb a variety of gases. Researchers at Notre Dame have pioneered
a technique using ionic liquids to separate CO2 from the flue gas discharged
by conventional power plants. The process is especially important to reducing
greenhouse gas emissions that contribute to global warming.
According to the National
Snow and Ice Data Center, summer ice covering the Arctic Ocean shrank to its
smallest size in more than a century. The decades-long shift in ice cover is
difficult to explain without accepting, at least in part, man’s
impact upon the environment. An increase in CO2 concentrations
in the atmosphere has also occurred since the onset of industrialization.
Today, CO2 concentrations have reached
approximately 380 parts per million, a number that’s
expected to rise to 500 or more parts per million by 2050.
Current
technologies for separating CO2 do so at a 30 percent energy penalty, meaning
that 30 percent of the energy generated is lost in the separation process. The
Notre Dame method offers the potential to significantly reduce this penalty and
has garnered considerable national attention. Funding for ionic liquids research
at Notre Dame over the last five years has exceeded $5 million.

Nuclear
power does not rely on fossil fuels and creates no greenhouse gases
that need to be sequestered. Yet nuclear power produces only 20 percent
of the nation’s
electricity. While countries like France and Japan have embraced nuclear
power, the U.S. has been reluctant to build more nuclear power plants.
Part
of President Bush’s Advanced Energy Initiative, the Global Nuclear
Energy Partnership teams the U.S. with supplier and user nations. Its
goals include development of a new generation of power plants; new
technologies that would recycle spent fuel, reducing the volume of
waste that would need to be stored in a geological repository; new
small-scale reactors for developing countries; and enhanced safeguards
that would make it more difficult to “divert
nuclear materials or modify systems without immediate detection.”
The
biggest problem with nuclear fission is what to do with the radioactive
waste. Where should it be stored? How should it be stored? And, how
long can it be safely stored before leaching into the environment?
Notre
Dame researchers, supported by a grant from the National Science Foundation,
have pioneered the identification of new compounds of uranium and other
radioactive nuclides, which will help them predict the mobility of
these compounds in the environment. Working with the Department of
Energy, they are also studying how radioactive materials would act
in a geological repository, such as the Yucca Mountain facility.

Can
energy supplies from clean coal technologies or nuclear power meet
the needs of a growing population? Or are other solutions needed? From
1900 to 2006, the world’s population more than quadrupled. During
the same time period, energy consumption increased more than 16-fold.
Today, there are 6.5 billion people who need energy to survive. By
2050, there may be 10 billion demanding their piece of the energy pie,
except they will have fewer resources from which to draw.
The options
open to them will likely be a combination of carbon-neutral energy
(clean coal technologies if commercially viable), nuclear power (which,
if used to address the entire projected need, would require the construction
of a new nuclear power plant every day for the next 50 years), and
renewable energy sources, such as hydropower, geothermal power, wind,
biomass, and solar energy. Unlike fossil fuels, renewable energy sources
never run out.
Accounting for 20 percent of the world’s electricity,
hydropower depends on the volumetric flow of the water and the height
from which it descends. One of the main advantages of hydrosystems
is that they do not require fuel. Without a doubt the most identifiable
source of hydroelectric power in North America is Niagara Falls. In
1893 water was first diverted from the Canadian side of the falls to
generate electricity, and a 2,200 kilowatt plant was built just above
the Horseshoe Falls. Today, approximately 2 million kilowatts of electricity
are generated from a number of sites along the Canadian side of the
falls. On the American side, additional power plants generate more
than 2.4 million kilowatts.
The world’s largest producer of hydropower,
Canada generates more than 70 percent of its electricity from hydroelectric
sources. Austria produces 67 percent, Iceland produces 83 percent,
and Norway produces virtually all of its electricity using hydrosystems.
Another
source of renewable energy is geothermal power. The world’s first
geothermal power plant was built in Larderello, Italy, in 1911. It
remained the only industrial producer of geothermal power until 1958.
Today, Larderello produces 10 percent of the world’s supply of
geothermal electricity, powering a million households. Although global
geothermal production has doubled in the last 20 years, geothermal
power trails hydropower production. It is also not a viable option
in many parts of the world, as not all geothermal areas have a high
enough temperature to produce steam.
According to the Global Wind Energy
Council, the global wind power market increased more than 40 percent
in 2005. Almost 25 percent of the new wind capacity was added in North
America, making it one of the world’s foremost implementers
of wind power. In fact, the Statue of Liberty and Ellis Island are
powered totally by wind energy.
Like other renewables, wind lessens
dependence on fossil fuels, such as coal and natural gas, provides
clean energy, and has the potential to support additional economic
development. The wind turbines, which can stand alone or connect to
a utility grid, operate up to 100 meters above the ground, where they
intercept faster, less turbulent air. When operating at peak power,
wind farms in the U.S. are expected to generate 25 billion kilowatt-hours
of electricity in 2006.
Biomass fuels (energy stored in organic matter)
provide three percent of all of the energy consumed in the U.S. and
produce 9 percent of the world’s
energy needs. Wood, agricultural waste, municipal solid waste, sugar
or starch crops, grass straw, soy beans, and waste vegetable oil are
all sources for biomass fuels, which can be converted to liquid fuels,
such as methanol and ethanol, as well as to heat and electricity. Although
a subject of great debate regarding the specific percentages involved,
it may take almost as much energy (most likely in the form of fossil
fuel) to produce one liter of biomass fuel as is obtained from burning
one liter of the fuel. Currently, only the sugar/carbohydrate content
of plants is used to produce biomass fuels; the higher the sugar/carbohydrate
content, the larger the ratio between the energy produced and the energy
consumed. So while this renewable energy source appears to be a promising
alternative to gasoline, there are many aspects in need of further
research — such as
using the whole plant, including its cellulose, or developing crops
specifically for use in biomass fuels — before these types of
fuels are commercially viable.
Solar power can be used for heating and
to produce electricity. In the simplest of terms, a solar cell converts
light from the sun into electricity. A photovoltaic cell is composed
of a semiconductor material that absorbs visible light and converts
the incident light into electricity. Though a clean and sustainable
source of energy, photovoltaic cells are not currently competitive
when compared to other options. However, the decreasing cost of production
and increasing demand for clean energy are likely to make photovoltaics
a viable option in the future.
University researchers are working to reconfigure photovoltaic cells using nanomaterials.
In particular, they are studying means by which cells and photocatalytic processes
can be made more efficient and less costly through the use of nanoparticles and
hybrid inorganic-organic materials. |
 |
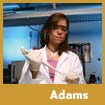 |
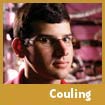 |
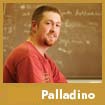 |
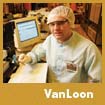 |
Undergraduates entering
the College of Engineering have many opportunities. Among the most
exciting are hands-on research experiences, particularly in the
field of energy. This year four students were selected to participate
in the Vincent P. Slatt Endowment for Undergraduate Research in
Energy Systems and Processes. Junior Laura
Adams, civil engineering
and geological sciences; senior David
Couling, chemical and biomolecular
engineering; senior Mark Palladino, electrical engineering; and
junior Peter VanLoon, computer science and engineering, were chosen
as Slatt scholars.
The Slatt Endowment was created by Christopher (B.S., EE ’80)
and Jeanine Slatt in honor of Vincent
P. Slatt (B.S., EE ’43),
the visionary incorporator of the National Rural Utilities Cooperative
Finance Corporation (CFC). Founded in 1969, CFC provides financing
for more than 1,050 electric cooperatives nationwide. These cooperatives
serve more than 39 million people (12 percent of all U.S. consumers)
and account for approximately five percent of electricity generating
capacity. The endowment recognizes and supports the energy related
research activities of undergraduates, from the use of fossil fuels
and nuclear and renewable energy sources to the development of more
efficient transportation and energy utilization systems.
This year’s
projects highlighted a wide range of topics. Adams studied factors
that could impact the release of radioactive materials from a nuclear
repository, such as the facility in Yucca Mountain, Nevada. Her adviser
was Peter C. Burns, the Henry J. Massman Jr. Chair of the Department
of Civil Engineering and Geological Sciences.
Making the most efficient
use of ionic liquids as industrial solvents, specifically for gas
separations, was the focus of Couling’s
research. Couling studied the equilibrium solubilities of different
mixtures of gases in a variety of ionic liquids to determine the
optimum mixture for gas solubility. His adviser was Joan
F. Brennecke, the Keating-Crawford Professor of Chemical and Biomolecular Engineering
and director of the Notre Dame Energy Center.
Palladino worked with
X. Sharon Hu, associate professor of computer science and engineering,
to address energy consumption in electronic systems. Preliminary
results of the project, which included a novel cache design, were
presented at the International Conference on Computer Aided Design,
a premier conference in the field of computing.
VanLoon explored the
feasibility of a new tunneling transistor that would require less
power to change the transistor from “on” to “off.” A
device built using such transistors would conserve energy. Working
on the nanoscale with adviser Alan C.
Seabaugh, professor of electrical
engineering, VanLoon employed electron beam lithography and other
processes available in the department’s nanofabrication facility. |
|

As
focused as the country seems to be on energy, or at least on gas prices,
energy ... having the resources to power cell phones, computers, airplanes,
grocery stores, and home heating systems ... has long been taken for
granted. People joke about the quality of life today compared to 40
or 50 years ago: “When I was growing up, we didn’t have
cable or iPods.“ They share stories about hauling firewood or
stoking coal stoves. They even suggest that if George Washington or
Abraham Lincoln were alive today they wouldn’t recognize the
country or know how to use most of the devices they would encounter.
The fact of the matter is that Washington and Lincoln would probably fare better
in the 21st century than today’s consumers would in the 1700s or 1800s — with
no Internet, no cell phones, no radio or television, no refrigeration or air
conditioning, no cars, and no cross-country flight service. Washington faced
a huge challenge at the birth of this nation; Lincoln confronted different issues
at its rebirth. They both used the tools available to them to help forge a better
country. Today’s engineers and scientists, working as stewards of knowledge
and the environment, are attempting to forge a new set of tools as they work
to better power the world.
For more information about energy research and education at Notre Dame, visit http://energycenter.nd.edu. |
 |
On May 3, 2006, during
an interview on NBC’s “Today” show, Rex W. Tillerson,
the chairman and chief executive officer of Exxon Mobil Corp.,
was questioned about the company’s record profits and their
relationship to skyrocketing gas prices. His response was pointed.
He said, “We work for the shareholder ... Our job is to make
the most money for them so their pension[s] are secure.” While
charging what the market will bear and returning a good profit
for investors are parts of any sound business plan, many would
argue that the bottom line is not the sole factor that should be
considered when dealing with energy in the 21st century.
From the
responsible use of a shrinking supply of fossil fuels to the economic
consequences and ethical implications of current energy policies,
today’s college students will face serious energy
challenges throughout their lives. In order to help students better
understand the challenges while appreciating that engineers cannot
operate in a vacuum, the College of Engineering introduced courses
relating to energy, society, and the climate in 2005.
The first course, “Energy
and Society,” is one of several
Engineering, Science, Technology, and Society courses offered. It
provides a comprehensive review of the role of energy in society
and covers a variety of social, economic, and political issues associated
with energy, as well as scientific and technical applications. Last
semester under the direction of Dean Frank
P. Incropera, the course
instructor, engineering and non-engineering undergraduates studied
different forms of energy, as well as the limitations of current
technologies. They reviewed the economic and environmental impact
of alternative energy sources, and they followed the actions of many
of the global players, such as the Organization of Petroleum Exporting
Countries (OPEC), Russia, China, and the United States. Most importantly,
through consideration of ethical and social justice issues, they
attempted to integrate their faith with decisions concerning future
energy utilization and development. For more information on “Energy
and Society” and other ESTS courses, visit http://www.nd.edu/~engineer/energy/ESTS.htm.
Another
course, “Energy and Climate,” addresses the magnitude
of world energy needs, quantifies the link between energy use and
climate cycles, and identifies the challenges of producing environmentally
friendly energy sources. “Part of what Notre Dame brings to
the table,” says Joan F. Brennecke, director of the Notre Dame
Energy Center and Keating-Crawford Professor of Chemical and Biomolecular
Engineering, is the integration of research and teaching with regard
to energy. It is vital for the next generation to understand how
we utilize energy and incorporate it into our daily lives, so that
they can make sound decisions about energy usage and energy policy.” Taught
by Brennecke and Mark J. McCready, professor and chair of the Department
of Chemical and Biomolecular Engineering, course topics include power
cycle analysis, atmospheric chemistry and climate modeling, coal,
biomass fuels, wind and hydroelectric power, weather cycles, and
nuclear energy. |
|
|
|