While X-rays have long identified
broken bones, emerging imaging technologies are being used to improve
the quality of images available for more accurate diagnoses and treatment,
assess radiation dosing, and find causes and cures for a variety
of diseases.
Angiography, arthrography,
chest X-ray, cholangiography, cholecystography, dental X-ray, mammography,
myelography, and pyelography ... are more than unpronounceable terms
in a medical dictionary. They are all X-rays — radiographic
(still) and fluoroscopic (real-time, moving) images — of different
parts of the body.
X-rays were
discovered by Wilhelm Röntgen in 1895. He called the new rays he found
X-rays because their nature was as yet unknown. What he did know was that through
the experiments he conducted sending the new rays (a new type of radiation)
through objects, even the human body, he could capture an image of the object.
|
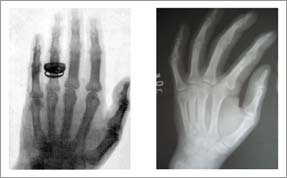 |
<< One of the earliest X-rays is on the
left with a more recent radiograph on the right. Although the modern
shot is sharper, the images are very similar. The first X-ray tubes
were very low power, requiring exposure (to the radiation) for several
minutes. Today, the exposure time is much lower, but there is still
tremendous potential for improving the image quality. |
Considered the father of diagnostic
radiology, Röntgen produced three papers on the subject and received
the first Nobel Prize in Physics for his discovery. In one of the papers,
Röntgen revealed the image of the first X-ray of the bones in
a living hand, his wife’s, and suggested the use of X-rays for
medical diagnostic work. Within a year X-rays of the stomach and other
organs were being used to identify and locate tumors, and within 10
years hospitals around the world were using X-rays to help diagnose
and treat patients. Physicians were finally able to see inside the
body without performing surgery.
“It’s hard to imagine the excitement this must have caused in the
scientific and medical communities,” says Ken D. Sauer, associate
professor of electrical engineering and director of the Engineering Honors Program, “even
though we can track the incredible and continued development of medical imaging
technologies, such as CTs, MRIs, and the creation of three-dimensional imaging,
and related equipment. The purpose of each innovation, of course, has been to
wring as much information as possible from an X-ray (scan) quickly and accurately
without harming the patient.”
X-rays are particularly helpful for viewing bones but can also identify some
diseases in soft tissue, such as lung cancer, pneumonia, or pulmonary edema.
The majority of soft tissue images, however, are better served through computed
axial tomography (CAT or CT scanning), magnetic resonance imaging (MRI), or ultrasound.
According to Sauer, the workhorse of tomography has been CT scanning. Much of
his research focuses on improving the methods for image reconstruction in these
types of scans. “We don’t work on the machine itself or design the
X-ray emitters,” he says. “We take the data that’s created
during the scan and apply very specific algorithms (part of a software package)
to help us better reconstruct the images. When these algorithms are successful,
they are built into special-purpose hardware, which increases the speed and accuracy
of image reconstruction.”
|
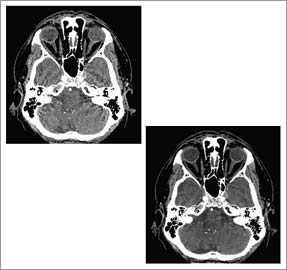 |
<< A
collaborative project with GE Healthcare and Purdue University that
focuses on developing statistically based tomographic reconstruction
algorithms for computed tomography has yielded much clearer images
than previously available. The conventional reconstruction method
is shown using a helical CT scan of the head, left photo, and the
advanced technique, developed by Ken D. Sauer, associate professor
of electrical engineering at Notre Dame; Jean-Baptiste Thibault (M.S.,
EE ’98; Ph.D., EE ’06), a CT scientist in the Applied
Science Laboratory at GE Healthcare; Charles A. Bouman, professor
of electrical and computer engineering at Purdue University; and
Jiang Hsieh, chief scientist in GE’s Applied Science Laboratory. |
Sauer has been developing statistically
based tomographic reconstruction algorithms for almost 20 years. In
the past five years he has noticed an increase of interest in lowering
the amount of radiation patients are exposed to during a procedure. “Lowering
the radiation a patient encounters during a scan means lower-quality
images, unless we do something to aid the reconstruction process. That,” he
says, “is the challenge.”
Radiation therapies for cancer treatment present different challenges for medical
imaging technologies. Intensity- modulated radiation therapy (IMRT), for example,
is designed to protect normal tissue through a series of treatment steps: organ
contouring, beam angle selection, dosage calculations, leaf sequencing, and delivery.
Organ/tumor contouring begins with a CT scan. The location and contours of the
target tumor area and surrounding tissue are determined via the CT images. Using
the information garnered during the contouring stage, a radiation dosage is calculated.
Leaf sequencing then helps identify the movements that should be taken by the
machine delivering the radiation, such as a linear particle accelerator, to assure
that the correct amount of radiation is distributed accurately to the tumor in
the minimum amount of time.
|
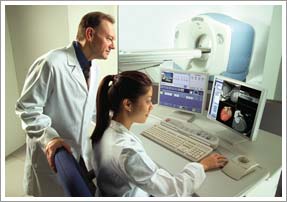 |
<< The algorithms developed through the
collaborative efforts of Notre Dame, GE Healthcare, and Purdue University
are designed to run on the GE Lightspeed VCT scanner, shown here. |
Researchers at Notre Dame,
in conjunction with researchers at the University of Iowa and the University
of Maryland School of Medicine, are addressing some of these steps. Danny
Z. Chen, professor of computer science and engineering, has
been working with Milan Sonka, professor of electrical
and computer engineering at the University of Iowa, on a National Institutes
of Health project to refine image segmentation (organ contouring). “Medical
imaging is a physician’s eye into the body,” says Chen. “Even
when you know the disease, you have to know exactly where it is located
to avoid damaging healthy tissue and vital organs. You need all of
this information before you can accomplish effective treatment.”
Chen and X. Sharon Hu, associate professor of computer science
and engineering and director of the department’s graduate studies program,
are also developing hardware-based methods to enhance image registration. One
of the major problems in medical imaging is that scans of the same area in the
same body do not always “match,” meaning it is not easy for a physician
to pinpoint one location from scan to scan. “This is very important,” says
Hu, “especially for mapping organs that move (change shape, such as the
heart and lungs), tracking the amount of radiation absorbed by a tumor, or assessing
the growth or reduction of a tumor.” Hu says there are many applications
outside of the medical field — such as the registration of satellite images,
but she and Chen have been concentrating on medical imaging. Whether for radiation
treatment or computer-aided surgery, fast and accurate image registration is
vital.
But achieving quick and precise image registration is not easy. Chen and Hu are
employing programmable hardware to assist in the task. Boards based on programmable
hardware (e.g., a field programmable gate array — FPGA) can be plugged
into a computer to greatly improve the accuracy and speed of image registration. “We
can buy the chips and build the board ourselves,” says Hu, “but for
research purposes, we purchase the programmable board, develop the bitstream
data (code) ourselves, and download that information onto the board, which plugs
into the computer and works like dedicated hardware.” This allows Chen
and Hu to test their work with the speed, but not the cost, of a dedicated system.
As a result of their efforts, the group has successfully developed an FPGA-based
solution for radiation dose calculation.
|
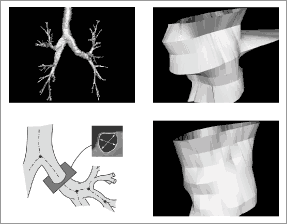 |
<< Notre Dame’s
Danny Z. Chen, professor of computer science and engineering, and
Milan Sonka, professor of electrical and computer engineering at
the University of Iowa, have applied for a patent on the approach
they have developed to refine the organ contouring process. Their
approach is faster, more accurate, and has solved problems once considered
computationally intractable when imaging three-dimensional objects.
From bottom left, clockwise, the photos show the cross-section of
the artery being scanned, the outcome of the scan employing the commonly
used 2-D algorithms, and the result of the new 3-D approach designed
by Chen and Sonka. |
Dr. Cedric Yu, professor
of radiation oncology and chief of physics at the University of Maryland
School of Medicine, and Bo Zhou, the postdoctoral
research associate working with Chen and Hu, have been testing the
Notre Dame algorithms on the machines in the Department of Radiation
Oncology at Maryland, with promisingresults. The Notre Dame researchers
and Dr. Yu have filed for two patents for radiation treatment, and
are working on additional projects to employ the imaging software and
hardware in the planning of cancer surgeries.
Chen and the Notre Dame computational medicine group also developed new leaf
sequencing algorithms and software called SLS. The algorithms, based on graph
theory models and computational geometry techniques, are producing IMRT plans
that reduce the treatment time by more than 60 percent while providing higher
quality images. In fact, the software is being used in the University of Maryland
Medical Center and the Helen P. Denit Cancer Center in Montgomery General Hospital
in Olney, Md., where it has aided the treatment of hundreds of patients since
2003.
Chen and Yu are also working on the challenges involved in image-guided surgeries,
monitoring the surgery in real-time so the surgeon can “see” not
only where the organs are but also confirming that radiation, if it is being
applied, is hitting the target areas and not healthy tissue. “We are using
another novel method to solve these problems,” says Chen, “a marriage
of computer science and medicine, that we hope will form the cornerstone for
more effective treatment.” |
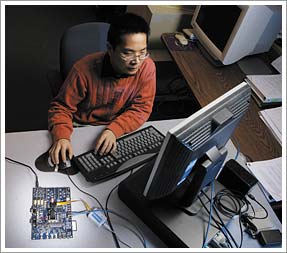 |
<< Bo
Zhou, a postdoctoral research associate working with X. Sharon Hu
and Danny Z. Chen, tests the programmable board the Notre Dame team
is using to address challenges in medical imaging technologies. In
a related project, a hardware-based radiation dose calculation system
developed by Hu and Chen, together with Kevin M. Whitton (M.S., CSE ’06)
and Dr. Cedric Yu at the University of Maryland, has been licensed
by Prowess, Inc., an international provider of medical software products
and services for the radiation therapy community. The new technology,
which performs the calculation on a programmable chip, alleviates
the bottleneck in radiation treatment planning. |
|