Hydrogen Generation
Background
As solar power gains in popularity, a question arises: how will power be provided at night? Hydrogen gas is one option for an energy storage medium. It is relatively simple to handle, and it can be utilized in combustion engines or fuel cells. Currently, about 95% of hydrogen produced is synthesized by methane reforming, where methane gas is converted to CO and H2. This process is energy negative, meaning that more energy is spent generating the hydrogen (plus the energy that could be generated by simply burning the methane) than can be recovered by burning the hydrogen. Obviously, this is not sustainable if we seek to move to renewable resources. Consequently, it is desirable to produce hydrogen from a renewable resource like solar power.
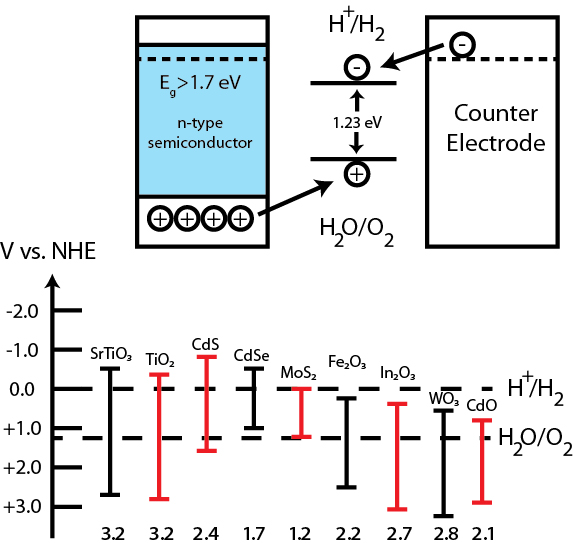
Energy diagram showing the conduction band and valance band potentials needed for a suitable photocatalytic water splitting system (above) along with the band positions of some common semiconductors (below).
Nature relies on photocatalysis to store energy, and we can follow its example to produce hydrogen from water. Many photocatalysts have been shown to photocatalytically split water into hydrogen and oxygen using only sunlight. There are three primary requirements for a photocatalyst to split water: 1) the photocatalyst must have a band gap of at least 1.23 eV (the thermodynamic potential for water splitting), although overpotentials due to slow reactions and resistance in the system make the required band gap closer to 1.7 or 1.8 eV; 2) the conduction band of the photocatalyst must be more negative than the hydrogen evolution potential (0 V vs. NHE at pH 0); 3) the valence band of the photocatalyst must be more positive than the oxygen evolution potential (+1.23 V vs. NHE at pH 0).
Many photocatalysts meet these requirements, though only a few actually show activity for water photolysis. Among these are materials like TiO2, SrTiO3, and La:NaTiO3. The major downside of these materials, however, is that they only absorb light in the ultraviolet region of the solar spectrum. More recently, materials like Fe2O3, WO3, GaZnON, and BiVO4 have been explored as potential photocatalysts.
In our lab, we are working on ways to produce hydrogen in a purely photocatalytic system that uses common materials and can be operated continuously. To accomplish this, we've developed a "reverse" fuel cell system. Traditionally, fuel cells combine hydrogen and oxygen to generate electricity, producing water as a product. In the "reverse" fuel cell, water is the feedstock, and oxygen and hydrogen are generated as products. While our work thus far has focused on single photocatalysts, the fuel cell can be modified to use two photocatalysts, with each performing one of the half-reactions.
Check out these links for more info!
- The Role of IrO2 in Mediating Hole Transfer at the TiO2 Interface - A slideshare webinar on a recent paper on photocatalyic hydrogen generation using a TiO2/IrO2 system.
- The Hydrogen Economy - Wikipedia entry on the Hydrogen Economy - the potential for using hydrogen as the main energy carrier.
- Natural Gas Reforming - Steam reforming of mathane to form hydrogen currently accounts for 95% of US hydrogen production.
Our Research Focus
- Develop continuous photocatalytic water splitting systems
- Elucidate the charge transfer interactions in semiconductor-catalyst systems
Recent Progress
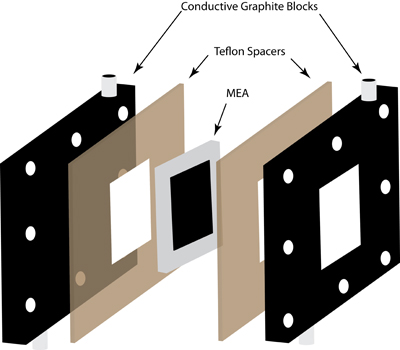
- Discovered a parasitic mechanism of the catalyst IrO2 (used as an electrocatalyst in industry) when applied to photocatalytic systems using TiO2
- Demonstrated the concept of continuous hydrogen generation utilizing the "reverse" fuel cell
A schematic of a water splitting cell, or reverse fuel cell, designed for the continuous production of hydrogen and oxygen upon illumination through outer window.
Select Publications
421. Manipulation of Charge Transfer Across Semiconductor Interface. A Criterion that Cannot be Ignored in Photocatalyst Design. Kamat, P. V. J.Phys. Chem. Lett. 2012, 3 ASAP. (Perspective article)
415. Role of Water Oxidation Catalyst, IrO2 in Shuttling Photogenerated Holes Across TiO2 Interface Meekins, B. H.; Kamat, P. V., J. Phys. Chem. Lett. 2011, 2, 2304-2310.
385. Fuel Cell Geared in Reverse. Photocatalytic Hydrogen Production using a TiO2/Nafion/Pt Membrane Assembly with No Applied Bias Seger, B.; Kamat, P. V. J. Phys. Chem. C 2009, 113,18946–18952. NDRL 4820