Light Energy Conversion
Background
Light as Energy
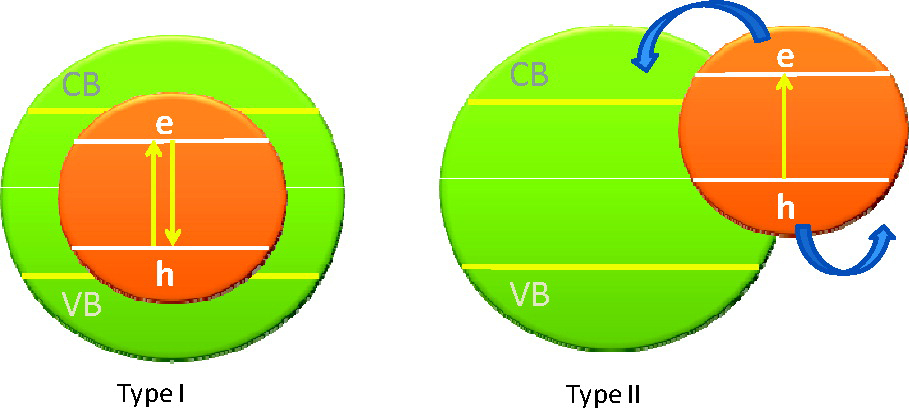
Figure. Designing a system with a Type I band alignment (left) promotes radiative recombination (i.e. when the excited electron loses energy it gives off a photon) which can be useful in LEDs. A Type II band alignment (right) can provide long lived excited electrons which can be used for photocatalysis or solar cells.
All light is made of photons, small "packets" of energy, the energy of which depends on the color, or wavelength, of the light. When a material absorbs a photon of light, the energy of the photon is transferred to an electron in the material, we call this an excited electron. This process is happening all of the time in every colored object that we see - the dye molecules in a red shirt are constantly absorbing blue, green, and yellow photons and reflecting red photons back to our eyes. In most objects, soon after the photon's energy is transferred to an electron, the electron loses that energy as heat, but, if the system is designed correctly, we can use that excited electron to do work. Depending on the system, these excited electrons can be driven through a wire as in a solar cell, or they can be used directly to perform a reaction as in solar hydrogen production.
Harvesting Light with Nanostrucures
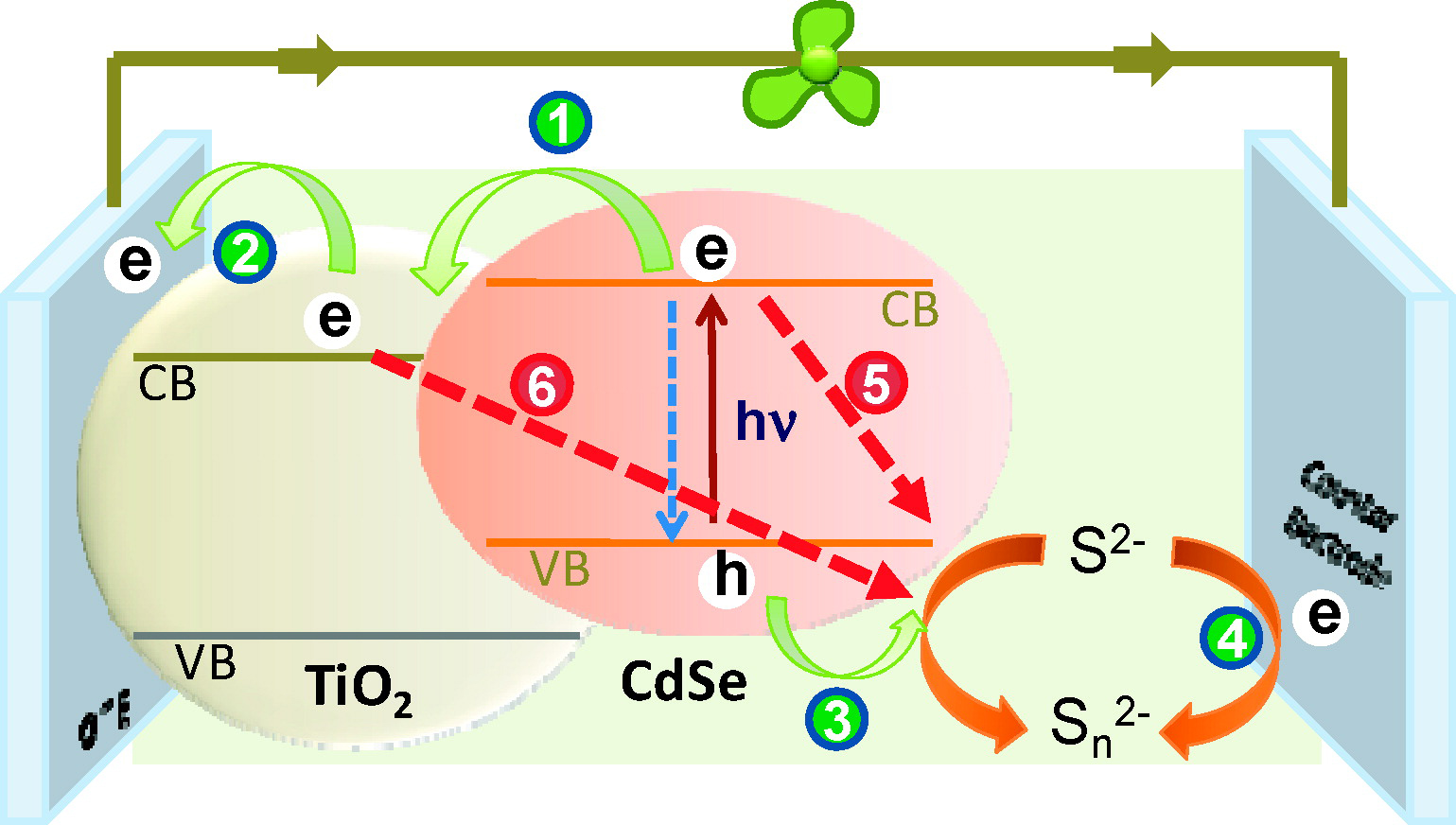
Figure. Schematic of the different charge transfer pathways in a Quantum Dot Solar Cell.
During the last decade, nanomaterials have emerged as the new building blocks to construct these types of light energy harvesting assemblies. Organic and inorganic hybrid structures that exhibit improved selectivity and efficiency towards catalytic processes have been designed. Size dependent properties such as size quantization effects in semiconductor nanoparticles and quantized charging effects in metal nanoparticles provides the basis for developing new and effective systems. These nanostructures provide innovative strategies for designing next generation energy conversion devices. Recent efforts to synthesize nanostructures with well defined geometrical shapes (e.g., solid and hollow spheres, prisms, rods, wires) and their assembly as 2- and 3- dimensional assemblies has further expanded the possibility of developing new strategies for light energy conversion.
There are three major ways that one can utilize nanostructures for the design of solar energy conversion devices. The first is to mimic the photosynthesis with donor-acceptor molecular assemblies and clusters. The second is the semiconductor assisted photocatalysis to produce fuels such as hydrogen. The third, and most promising category, is the nanostructure semiconductor based solar cells.
Check out these links for more info!
- Solar Energy - Beyond the Hype - Webinar by Dr. Prashant Kamat on the clean energy challenge and solar energy.
- A Delicious Solar Cell - A solar cell made from Doughnuts and Tea by former group member Blake Farrow (Youtube video)
Our Research Focus
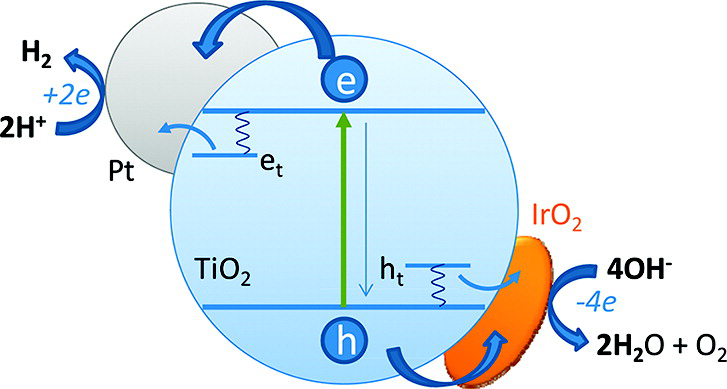
Figure. Design of a photocatalytic water splitting system utilizing Pt/TiO2/IrO2 where TiO2 is used as the light absorber, Pt as the hydrogen evolution catalyst, and IrO2 as the oxygen evolution catalyst.
- Design heterogeneous assemblies consisting of carbon nanostructures (graphene, fullerenes, carbon nanotubes, etc.), semiconductor nanostructures, metal nanoparticles and sensitizing dyes for harvesting light energy
- Elucidate the interfacial charge transfer processes in semiconductor/sensitizer systems
- Design nanostructured assemblies for the direct splitting of water into hydrogen and oxygen gas
Recent Progress
- Investigated the photoactivity of metal clusters
- Developed panchromatic systems employing multiple semiconductors and semiconductor/dye composites
- Elucidated electron and hole transfer reactions in a variety of semiconductor systems
- Applied knowledge of photophysical processes to the fabrication of higher efficiency solar cells
Select Publications
449. Excited State Behavior of Luminescent Glutathione Protected Gold Clusters Stamplecoskie, K. G.; Chen, Y.-S.; Kamat, P. V. J. Phys. Chem. C 2013, ASAP.
445. CdS Nanowire Solar Cells: Dual Role of Squaraine Dye as a Sensitizer and a Hole Transporter Choi, H.; Kamat, P. V. J. Phys. Chem. Lett. 2013, 4, 3983–3991.
443. Trap and Transfer. Two-Step Hole Injection Across the Sb2S3/CuSCN Interface in Solid State Solar Cells. Christians, J. A.; Kamat, P. V. ACS Nano 2013, 7 (9), 7967–7974.
442. Quantum Dot Surface Chemistry: Ligand Effects and Electron Transfer Reactions. Hines, D. A.; Kamat, P. V. J. Phys. Chem. C 2013, 117 (27), 14418–14426.
441. Metal-Cluster-Sensitized Solar Cells. A New Class of Thiolated Gold Sensitizers Delivering Efficiency Greater Than 2%. Chen, Y.-S.; Choi, H.; Kamat, P. V. J. Am. Chem. Soc. 2013, 135 (24), pp 8822–8825.
435. CdSe Nanowire Solar Cells Using Carbazole as a Surface Modifier. Choi, H.; Kuno, M.; Hartland, G. V.; Kamat, P. V. J. Mater. Chem. A 2013, 1, 5487-5491.
433. Tandem-Layered Quantum Dot Solar Cells: Tuning the Photovoltaic Response with Luminescent Ternary Cadmium Chalcogenides. Santra, P.; Kamat, P. V. J. Am. Chem. Soc. 2013, 135 (2), 877–885.
430. Realizing Visible Photoactivity of Metal Nanoparticles: Excited-State Behavior and Electron-Transfer Properties of Silver (Ag8) Clusters. Chen, W. T.; Hsu, Y. J.; Kamat, P. V. J. Phys. Chem. Lett. 2012, 3, 2493–2499.
428. Photoinduced Surface Oxidation and Its Effect on the Exciton Dynamics of CdSe Quantum Dots. Hines, D. A.; Becker, M. A.; Kamat, P. V. J. Phys. Chem. C 2012, 116 (24),13452–13457.
423. Synchronized energy and electron transfer processes in covalently linked CdSe-squaraine dye-TiO2 light harvesting assembly. Choi, H.; Santra, P. K.; Kamat, P. V. ACS Nano 2012, 6 (6), 5718–5726.
421. Manipulation of Charge Transfer Across Semiconductor Interface. A Criterion that Cannot be Ignored in Photocatalyst Design. Kamat, P. V. J.Phys. Chem. Lett. 2012, 3 (5), 663–672. (Perspective article)
417. Supersensitization of CdS Quantum Dots with NIR Organic Dye: Towards the Design of Panchromatic Hybrid-Sensitized Solar Cells Choi, H.; Nicolaescu, R.; Paek, S.; Ko, J.; Kamat, P. V. ACS Nano 2011, 5, 9238–9245.